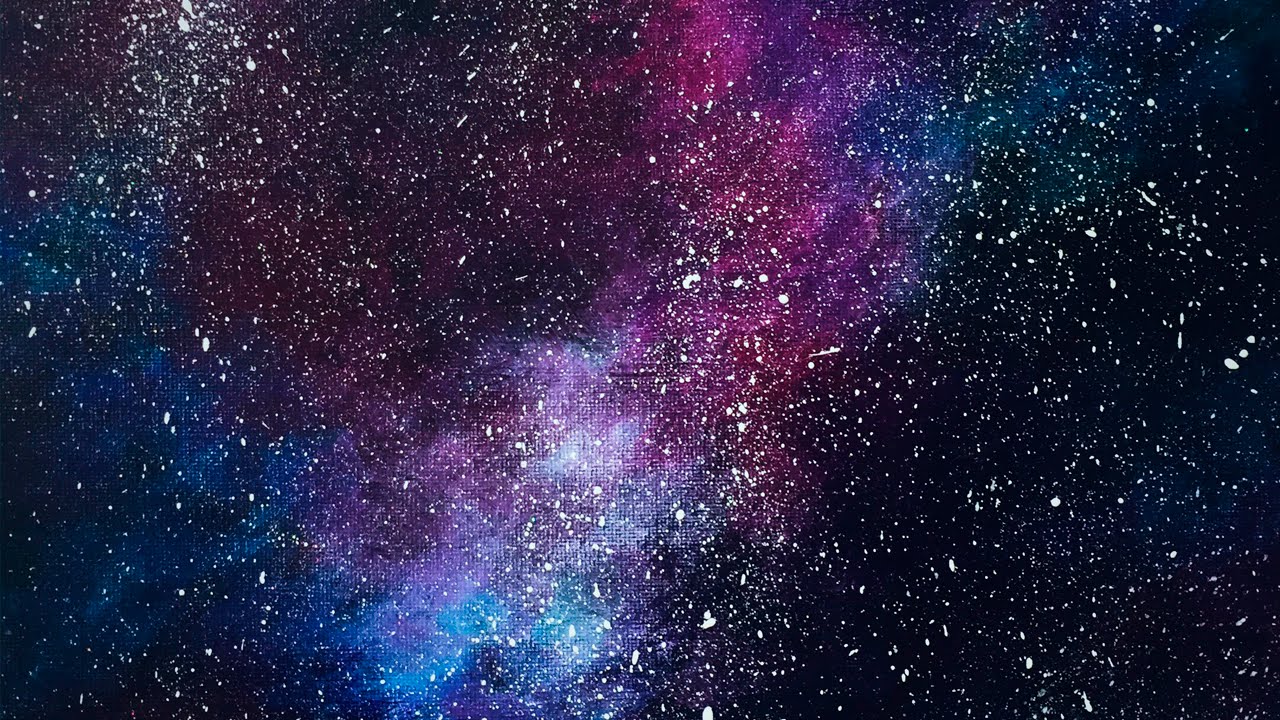
Why matter exist? Why antimatter exist? Universe should not exist, scientists reveal
Before Stephen Hawking died on March 14, he stated that the universe came into existence from ‘nothing’. Questions related to how the universe came into existence? Why matter exist? Why antimatter exist? And others have been the basic principles of metaphysics. With advancement in technology and scientific equipment, researchers have started applying scientific methods to answer these questions that have become a part of the cosmic conundrum. According to a report published in CNN, there is something because of which, we are here. If it was opposite, we wouldn’t be here. So the questions arise are, Why the universe is not a featureless void? Why universe does have matter rather than just energy. There are a plethora of theories and hypothesis obtained by scientists over the years of which, some are explained below.
Researchers at the Sanford Underground Research Facility started working on a project entitled ‘Majorana Demonstrator’ where the site is located near Lead, South Dakota. The researchers started working on a project that involves placing a rare isotope of germanium 65 pounds in a quantity that has been cooled down to the temperature that it has started liquefying air. Then, they place their scientific equipment nearly one mile deep to gauge the answers whether why the universe is made up of matter exclusively. Talking about the theory that literally contradicts the universe as we observe. To put things into context, Standard Model is a theory according to which, there are an equivalent amount of matter and its antimatter which is a substance that complements a matter.
Theoretically, when matter and antimatter are fused together, it results in 100% conversion in energy. In fact, a paper clip made up of the matter is fused with a paper clip made of antimatter, it will result in energy far greater than the atomic bomb that was detonated in Hiroshima, Japan. Since, our universe was formed due to Big Bang as per modern cosmology as per which, energy explosion resulted in the equal amount of matter and antimatter that must have been resulted. But where is antimatter? Whatever we observe around us, in the universe, it is all made up of matter while the existence of antimatter has become a mystery ever since it was first discovered in 1964 and it is still being studied.
A theory believes that while our early universe was taken shape, a slight anomaly or asymmetry bent the laws of nature that resulted in different properties of matter and antimatter. It is believed that for every one billion antimatter subatomic particles formed in the Big Bang, it created a one billion and one matter. As matter and antimatter annihilate itself, the One billion matter and antimatter particles annihilated itself resulting it a leftover of matter. The leftover matter formed the universe and all the celestial bodies that we observe now.
But this isn’t the only explanation available in the scientific world. In a different theory, leptons which are a class of subatomic particles familiar to electrons are found revolving around atoms. However, there is a lesser-known class of leptons and neutrinos that emit nuclear radiation through the process called beta decay. As per the process, nuclear radiation is produced when neutrons present in an atom decays to found in neutrino, electron, and proton. These particles have extremely weak interaction and thus, difficult to study or even detect. Talking about neutrino, there could be matter and antimatter neutrino present in the universe which is a mystery and needs further investigation.
After years of research on neutrino, scientists know that there are both types of neutrinos, however, the question is whether these are formed together or they are different. It is difficult to recognize both the type of matter since these are like identical twins where both look exactly the same. Apart from beta decay, scientists are also contemplating and experimenting with double beta decay, neutrinoless double beta decay and other sorts of subatomic phenomena before they can find the results on why everything in the universe is made exclusively of matter and not antimatter.
The full statement from the Sanford Underground Research Facility follows below:
If equal amounts of matter and antimatter had formed in the Big Bang more than 13 billion years ago, one would have annihilated the other upon meeting, and today’s universe would be full of energy but none of the matter needed to form stars, planets, and life.
That means the very existence of matter suggests something is wrong with Standard Model equations describing symmetry between subatomic particles and their antiparticles.
“The excess of matter over antimatter is one of the most compelling mysteries in science,” said John Wilkerson of ORNL and the University of North Carolina, Chapel Hill. Wilkerson leads the Majorana Demonstrator, which involves 129 researchers from 27 institutions and 6 nations. “Our experiment seeks to observe a phenomenon called ‘neutrinoless double-beta decay’ in atomic nuclei. The observation would demonstrate that neutrinos are their own antiparticles and have profound implications for our understanding of the universe. In addition, these measurements could provide a better understanding of neutrino mass.”
Sanford Underground Research Facility built the laboratory space that houses the experiment and provides support for the daily operations. Majorana, the first physics experiment to begin operations on the 4850 Level, began growing ultrapure copper for its highly sensitive experiment in 2011.
“This is great news for the Majorana Demonstrator collaboration,” said Mike Headley, executive director for South Dakota Science and Technology Authority and laboratory director for Sanford Lab. “Their trust in us helped lead the way for us to become a world-class physics research facility.”
Headley noted that Sanford Lab Staff members are responsible for helping with daily logistics that keep the experiment clean and running. “I’m very proud of the work this team has done to help Majorana reach this major milestone.”
In a 2015 report of the U.S. Nuclear Science Advisory Committee to the Department of Energy and the National Science Foundation, a U.S.-led ton-scale experiment to detect neutrinoless double-beta decay was deemed a top priority of the nuclear physics community. Nearly a dozen experiments have sought neutrinoless double-beta decay, and as many future experiments have been proposed. One of their keys to success depends on avoiding background that could mimic the signal of neutrinoless double-beta decay.
That was the key accomplishment of the Majorana Demonstrator. Its implementation was completed in South Dakota in September 2016, nearly a mile underground at the Sanford Underground Research Facility. Siting the experiment under nearly a mile of rock was the first of many steps collaborators took to reduce interference from background. Other steps included a cryostat made of the world’s purest copper and a complex six-layer shield to eliminate interference from cosmic rays, radon, dust, fingerprints and naturally occurring radioactive isotopes.
“If you’re going to search for neutrinoless double-beta decay, it’s critical to know that radioactive background is not going to overwhelm the signal you seek,” said ORNL’s David Radford, a lead scientist in the experiment.
There are many ways for an atomic nucleus to fall apart. A common decay mode happens when a neutron inside the nucleus emits an electron (called a “beta”) and an antineutrino to become a proton. In two-neutrino double-beta decay, two neutrons decay simultaneously to produce two protons, two electrons and two antineutrinos. This process has been observed. The Majorana Demonstrator Collaboration seeks evidence for a similar decay process that has never been observed, in which no neutrinos are emitted.
Conservation of the number of leptons—subatomic particles such as electrons, muons or neutrinos that do not take part in strong interactions—was written into the Standard Model of Physics. “There is no really good reason for this, just the observation that it appears that’s the case,” said Radford. “But if lepton number is not conserved, when added to processes that we think happened during the very early universe, that could help explain why there is more matter than antimatter.”
Many theorists believe that the lepton number is not conserved, that the neutrino and the antineutrino—which were assumed to have opposite lepton numbers—are really the same particle spinning in different ways. Italian physicist Ettore Majorana introduced that concept in 1937, predicting the existence of particles that are their own antiparticles.
The Majorana Demonstrator uses germanium crystals as both the source of double-beta decay and the means to detect it. Germanium-76 (Ge-76) decays to become selenium-76, which has a smaller mass. When germanium decays, mass gets converted to energy that is carried away by the electrons and the antineutrinos.
“If all that energy goes to the electrons, then none is left for neutrinos,” Radford said. “That’s a clear identifier that we found the event we’re looking for.”
The scientists distinguish two-neutrino versus neutrinoless decay modes by their energy signatures. “It’s a common misconception that our experiments detect neutrinos,” said Jason Detwiler of the University of Washington, who is a co-spokesperson for the Majorana Collaboration. “It’s almost comical to say it, but we are searching for the absence of neutrinos. In the neutrinoless decay, the released energy is always a particular value. In the two-neutrino version, the released energy varies but is always smaller than for neutrinoless double-beta decay.”
The Majorana Demonstrator has shown that the neutrinoless double-beta decay half-life of Ge-76 is at least 1025 years—15 orders of magnitude longer than the age of the universe. So it’s impossible to wait for a single germanium nucleus to decay. “We get around the impossibility of watching one nucleus for a long time by instead watching on the order of 1026 nuclei for a shorter amount of time,” explained co-spokesperson Vincente Guiseppe of the University of South Carolina.
Chances of spotting a neutrinoless double-beta decay in Ge-76 are rare—no more than 1 for every 100,000 two-neutrino double-beta decays, Guiseppe said. Using detectors containing large amounts of germanium atoms increases the probability of spotting the rare decays. Between June 2015 and March 2017, the scientists observed no events with the energy profile of neutrinoless decay, the process that has not yet been observed (this was expected given the small number of germanium nuclei in the detector). However, they were encouraged to see many events with the energy profile of two-neutrino decays, verifying the detector could spot the decay process that has been observed.
The Majorana Collaboration’s results coincide with new results from a competing experiment in Italy called GERDA (for GERmanium Detector Array), which takes a complementary approach to studying the same phenomenon. “The Majorana Demonstrator and GERDA together have the lowest background of any neutrinoless double-beta decay experiment,” said Radford.
The Majorana Demonstrator was designed to lay the groundwork for a ton-scale experiment by demonstrating that backgrounds can be low enough to justify building a larger detector. Just as bigger telescopes collect more light and enable viewing of fainter objects, increasing the mass of germanium allows for a greater probability of observing the rare decay. With 30 times more germanium than the current experiment, the planned one-ton experiment would be able to spot the neutrinoless double-beta decay of just one germanium nucleus per year.